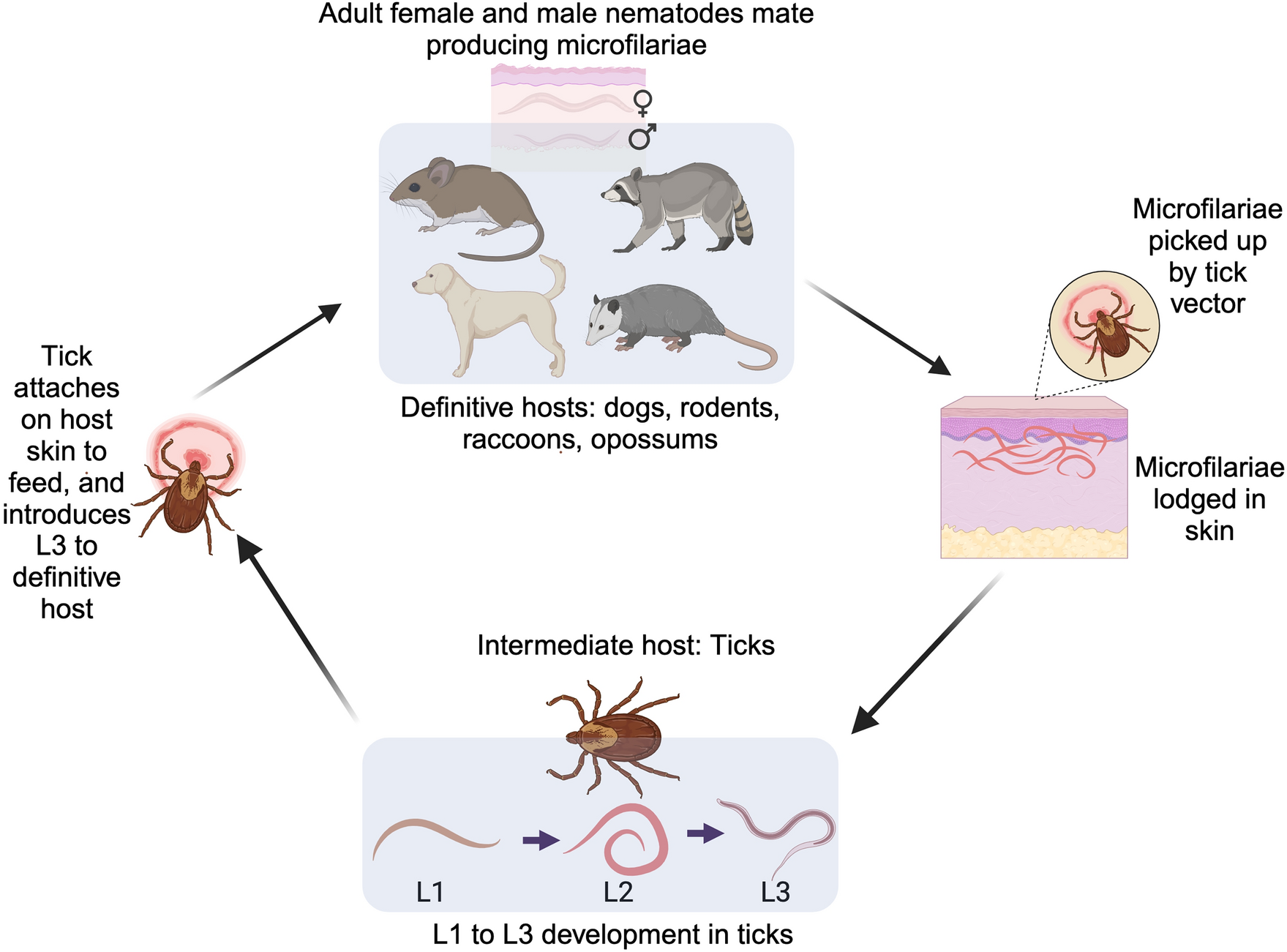
Advertisement
Parasites & Vectors volume 18, Article number: 50 (2025)
Metrics details
Ticks are well-known vectors of pathogens, including filarial nematodes, which can affect animal and human health. This review synthesizes current knowledge of filarial nematodes that utilize ticks as vectors, focusing on the Onchocercidae. Five genera of onchocercid filarial nematodes (Cercopithifilaria, Cherylia, Cruorifilaria, Monanema, and Yatesia) have demonstrated vector–parasite relationships with ticks, while Acanthocheilonema has only demonstrated molecular detection without confirmed vector competence. Vector competence has been experimentally proven for several species through documented development to infective stages. However, our understanding of tick-borne filarial nematodes remains limited owing to insufficient detection efforts and methodological challenges. Detection methods include traditional dissection, advanced imaging techniques such as microcomputed tomography (micro-CT), and molecular approaches, each with distinct advantages and limitations. To address knowledge gaps, future research should focus on conducting large-scale surveys of tick populations and wildlife to investigate vector competence, health impacts, and coinfection dynamics. Multi-disciplinary collaboration and community involvement in tick surveillance will advance our understanding of tick-borne filarial nematode ecology and epidemiology. Future research priorities include confirming vector competence where only molecular detection exists, investigating natural transmission patterns, and understanding the mechanisms influencing successful development in tick vectors. By addressing these knowledge gaps through systematic surveillance and experimental studies, we can better understand these complex host–parasite–vector relationships and their implications for animal health.
Ticks (Ixodida) are blood-feeding arthropods known to spread (i.e., vector) a range of pathogens of medical and veterinary importance [1, 2]. Ticks transmit a variety of disease-causing microbial taxa, spanning bacteria eliciting Lyme borreliosis, anaplasmosis, rickettsiosis, and ehrlichiosis [3,4,5]; viruses causing Powassan, Heartland, and tick-borne encephalitis infections [3, 6,7,8]; Babesia and Hepatozoon protozoan parasites causing babesiosis and hepatozoonosis, respectively [9,10,11]; and macroparasites, including filarial nematodes of medical and veterinary importance [12,13,14]. Filarial nematodes belonging to the superfamily Filarioidea Weinland, 1858 are further divided into two families: Filariidae Cobbold, 1864 and Onchocercidae Leiper, 1911. These nematodes have indirect life cycles, requiring arthropod intermediate hosts (biological vectors) to complete their development and transmission. Despite the diverse arthropod vectors utilized by filarial nematodes, only a few Onchocercidae species have been shown to use ticks as primary intermediate hosts [15]. Most Filarioidea rely on blood-sucking insects, such as mosquitoes, fleas, black flies, and biting midges, as primary vectors [16, 17]. Tick-transmitted filarioid nematodes show specific host associations; for example, Cercopithifilaria bainae infects domestic dogs and Monanema marmotae parasitizes groundhogs (Marmota monax) [12, 18]. Similarly, other arthropod-transmitted filariae demonstrate host specificity, including some filarioid species that are agents of neglected tropical diseases in humans, such as the black fly-transmitted Onchocerca volvulus and the mosquito-transmitted Wuchereria bancrofti in humans [19, 20].
Morphologically, filarial nematodes are elongated and threadlike, with long cylindrical bodies coated in a rigid cuticle with oral and anal openings, sensory papillae, and reproductive organs [16, 21, 22]. Nematode sexes are separated, with males and females sometimes being morphologically different; males may be shorter with coiled posterior ends, whereas females tend to be more elongated. Microfilariae are the initial larval stages of development of filarial nematodes, which develop in arthropod vectors into first-stage larva (L1), second-stage larvae (L2), and subsequently to third-stage larvae (L3), which is infective to the definitive host [16]. Vertebrate species typically serve as the definitive host for filarial nematodes, wherein adult nematodes attain sexual maturity and reproduce, with females producing fertilized eggs that hatch into microfilariae. Microfilariae can circulate throughout the vertebrate host body and are often ingested by arthropod vectors such as insects (Insecta) and acarines (Acari; ticks and mites) during blood-feeding activities, where the definitive host enables parasite reproduction and continued transmission [22,23,24].
The potential role of tick vectors in propagating filarial nematodes and affecting animal and human health remains understudied. Several studies have examined and identified filarial nematodes in both hard (Ixodidae) and soft (Argasidae) ticks [14, 25,26,27,28,29,30,31,32,33,34,35], thereby providing evidence that ticks can indeed vector filarial nematodes. Tick feeding strategies can significantly contribute to the biological transmission of filarial nematodes in several ways, including the duration of attachment, feeding preferences, and developmental stages. While slow-feeding ixodid ticks can remain attached to their hosts for several days or weeks, with some species feeding for up to 2 weeks [1], experimental studies by Brianti et al. [36] showed that extended feeding duration alone does not determine successful filarial nematode transmission. The typical development from microfilariae to infective L3 stage often takes longer than a single tick feeding period, usually around 30 days [36]. While this extended development time increases the likelihood of successful transmission to a new vertebrate host during subsequent feeding, reinfection of the same host during a single blood meal is unlikely. In addition, feeding patterns and host associations of ticks can play a crucial role in the life cycle of filarial nematodes [37]. For example, Ramos et al. [38] showed that Cercopithifilaria rugosicauda utilizes Ixodes ricinus nymphs to parasitize roe deer (Capreolus capreolus) in southern Italy. The timing of nymphal activity coincides with host availability, demonstrating how filarial transmission occurs through monotropic tick species—ticks that primarily parasitize specific host species.
While tick-borne filarial nematodes have been documented in various animal hosts, their impact on animal health remains poorly understood. Currently, there are no documented cases of tick-borne filarial nematodes affecting livestock production, and their economic significance, if any, requires further investigation. In companion animals, Cercopithifilaria spp. can infect dogs, with some naturally infected individuals developing dermal nodules, though these infections are rarely associated with clinical signs [23, 32, 36]. While treatments such as topical medications and corticosteroids are available, much remains to be understood about the pathogenicity and epidemiology of these infections [39].
In this review, we aim to synthesize and provide a comprehensive overview of the current knowledge on the global diversity of filarial nematode species that use ticks as vectors. Among the family Onchocercidae, development and transmission require arthropod intermediate hosts, with several genera specifically adapted to using ticks as vectors. Therefore, we focus on genera within Onchocercidae, reporting their known tick vectors and other arthropod vectors. In addition, using North America as a case study, we highlight significant gaps in the distribution and prevalence of tick-borne filarial nematodes and explore potential interactions between filarial nematodes and tick-borne pathogens and discuss their implications for infection dynamics and host immune responses. This review will cover the strengths and weaknesses of existing and current methods to detect filarial nematodes in tick vectors, emphasizing the need for targeted, large-scale research efforts focusing on known tick hotspots and risk interfaces. By synthesizing available information and critically assessing published literature, we aim to understand tick-borne filarial transmission among domestic and wildlife animals in natural ecosystems, with a focus on identifying knowledge gaps regarding these nematodes in North America to guide future research directions.
All vertebrate classes except fish serve as definitive hosts for filarial nematodes, enabling their development and transmission [40]. Vertebrate hosts harboring filarial nematodes allow the parasite to complete their life cycle, thus facilitating their transmission to tick vectors (Fig. 1). For instance, the life cycle of C. rugosicauda illustrates the synchronized timing between tick vectors and vertebrate hosts, which is essential for successful transmission. Development in I. ricinus takes 8 weeks for microfilariae to reach the infective L3 stage, followed by 24 weeks in roe deer for L3 to mature into adults [30, 38]. Adult nematodes produce microfilariae that concentrate in dermal tissues, coinciding with tick feeding periods and enabling continued transmission.
Transmission cycle of filarial nematodes in tick vectors and vertebrate hosts. The cycle begins when a tick vector introduces L3 larvae into the definitive host (e.g., dogs, rodents, raccoons, and opossums) through skin bites. Within the host, L3 larvae develop into adult nematodes that mate and produce microfilariae, which circulate in the skin or blood of the host. When a new tick feeds on an infected host, it ingests the microfilariae, which then develop into infective L1 to L3 larvae within the tick, completing the transmission cycle. Illustration created on Biorender.com
The efficiency of filarial nematode transmission between vertebrate hosts and tick vectors may depend on host–vector compatibility. The availability of compatible hosts and vector feeding preferences may determine the geographic distribution of vector-borne helminths. For instance, domestic dogs serve as efficient reservoirs for filarial nematodes by simultaneously maintaining long-term infections with multiple species and their ability to sustain infections, which makes them consistent sources of microfilariae for brown dog ticks throughout the vector active season [14, 36].
Early studies by Bain [28], Bain et al. [35], and Beaver and Burgdorfer [29] showed that the hindgut, hemocoel, fat cells, and salivary gland ducts of ticks provide a favorable environment for the development of certain filarial nematodes. In these cases, ticks ingest microfilariae when feeding on an infected vertebrate host [28] (Fig. 1). These microfilariae then migrate to specific tick tissues to find suitable conditions for growth and transformation into infective larvae. For example, many microfilariae migrate from the tick midgut to the hemocoel (i.e., body cavity), where they molt into L1, L2, and subsequently into infective L3, a process that may take 30 days [36]. L3 larvae have been documented in tick salivary glands [13, 36]; however, their transmission to vertebrate hosts via salivary glands is yet to be established. As ticks feed for several days, they regurgitate saliva into the bite site, potentially countering host hemostasis and inflammatory responses [22, 41,42,43]. Tick feeding can potentially deliver L3 larvae into the dermal skin layers of the host, setting off local reactions and enabling nematode transfer [18] (Fig. 1).
The concentration of microfilariae in the skin of infected vertebrates near common tick attachment sites (such as the ears) implies a specific attraction of filarial nematodes to chemical substances in tick saliva, as demonstrated by Ko [18]; a similar mechanism was proposed by Moorhouse [43]. If broadly applicable, this attraction could enhance the probability of microfilariae being ingested by the tick vector during blood feeding, facilitating their transmission to the vector and subsequently to new susceptible vertebrate hosts (Fig. 1). The specific vertebrate tissue harboring these transmitted microfilariae (e.g., skin or blood) corresponds to the feeding strategies of the arthropod vector on hosts [44]. For instance, microfilariae inhabiting subdermal skin layers may be ingested by ticks during their blood-feeding process. Alternatively, some circulating blood-borne microfilariae can be consumed through vessel lacerations by arthropod vectors such as mosquitoes and other flies that feed on blood using anticoagulant strategies. Thus, vertebrate microfilariae niche accessibility aligns with vector hematophagous behavior, which propagates initial establishment within intermediate hosts [45].
The life cycles of filarial nematodes within arthropod vectors are initiated when blood-feeding uptake introduces vertebrate-derived microfilariae into the vector system. The complex process by which nematodes develop from microfilariae to L3 within ticks involves multiple stages that occur in specific organs and tissues, with translocation through the stomach walls [22] (Fig. 1). While the role of saliva in tick-feeding has been well documented in tick-borne pathogens [46, 47], knowledge gaps exist on how filarial nematodes interact with and potentially impact tick organs. As microfilariae and developing larvae migrate through various tick tissues—from gut epithelium to hemocoel and salivary glands—they may affect tissue integrity and function. The efficiency of L3 from tick-to-host transfer could depend on these tissue interactions; aspects of this process remain elusive and require further investigation to comprehend the mechanisms at play.
Once in the definitive host, the infective L3 typically molts and migrates. Some L3 may move from the skin via lymphatic vessels to other vertebrate host tissues to continue development. Others may remain in the skin to mature into adults that mate and produce microfilariae, enabling acquisition by new ticks to perpetuate further transmission cycles [32] (Fig. 1). The immunogenic nature of incoming L3 larvae may influence the establishment of infection in the vertebrate host and host immune defenses at bite sites or downstream migration routes can impede nematode survival. Deciphering the fundamental processes governing these multifaceted vertebrate–tick–parasite associations may necessitate a deeper understanding of the complex interaction and impact on the circulation of filarial nematodes, as this affects host health.
Detecting and screening tick vectors for filarial nematode infections is essential for assessing the prevalence of these nematodes in tick populations, identifying potential risk areas for transmission, and developing targeted control strategies [14, 36]. Various techniques, including dissection [36, 38], histopathological analyses [14], and molecular approaches, such as polymerase chain reaction (PCR) and DNA sequencing [12, 33] (Table 1), can be used to assess the status of tick infection with filarial nematodes. These methods differ in sensitivity, specificity, and practicality, making it essential to consider the strengths and weaknesses of each approach when designing studies or surveillance programs.
Dissection and microscopic examination of ticks represent a classical means of investigating filarial infection and developmental progression within different tick tissues (Fig. 2A). Using these methodologies, filarial nematodes have been observed in hard tick species, including Amblyomma cajennense [31], Haemaphysalis flava, H. japonica [48], Ixodes cookei [18, 49], I. scapularis [29], and I. ricinus [30, 35, 38, 50]. Soft ticks, such as Ornithodoros tartakovskyi [51] and O. talaje [52], have also been found to carry filarial nematodes. Initial investigations into filarial nematode infections in ticks relied upon microscopic visualization methods for identification and morphological diagnosis. While microscopy and dissection techniques provided early verification that ticks harbor filarial nematodes, these methods have limitations in species identification. Morphological identification of filarial larvae is challenging owing to similar anatomical features among species and cryptic species complexes [53], particularly in early developmental stages. Therefore, while genus-level identification is possible through microscopy and dissection, molecular methods are typically needed for species-level determination.
Complementary approaches for detecting and characterizing filarial nematodes in tick vectors. (A) Classical methods involve invasive techniques such as dissecting and microscopically examining excised tick tissues to visualize and quantify filarial nematodes; (B) noninvasive imaging techniques, such as X-ray micro-computed tomography (micro-CT), allow for the visualization and localization of filarial nematodes within tick samples without dissection, generating high-resolution two-dimensional (2D) and three-dimensional (3D) images; (C) molecular techniques, such as DNA/RNA extraction (often requiring the destruction of the sample), followed by PCR, sequencing, or next-generation sequencing and computational analysis, enable the identification and characterization of filarial nematodes at the nucleotide level. Illustration created on Biorender.com
Patton et al. [54] established comprehensive protocols for dissecting tick organs and tissues, and for the collection of biofluids (i.e., hemolymph, saliva), enabling examination of tick internal structures for the presence of pathogens. These methods, later applied by Santos et al. [55] to study filarial nematodes, can be adapted to target specific tissues where these parasites develop, facilitating visual identification of larvae within ticks. Studies have demonstrated the presence of filarial nematodes in tick organs, such as the hindgut, hemocoel, fat cells, and salivary ducts [18, 29, 35, 36]. Controlled infection experiments have directly validated the ability of specific tick genera, including Amblyomma, Ixodes, and Rhipicephalus, to effectively acquire and transmit filarial nematodes [18, 31, 36, 49, 56, 57]. Despite being constrained by morphological assessment limitations and operator expertise, adopting standardized dissection and preparation protocols would result in more consistent documentation of tissue distributions and quantification of tick-borne filarial burden. Thus far, no definitive reports have explicitly validated the precise anatomical locations occupied by developing L1–L3 filarial nematodes inside tick vectors. Expanding confirmation of occupied tick niches through other methods, such as targeted imaging and molecular approaches, may enable tracking of filarial occupation across tick tissues, thereby elucidating vector–parasite interface dynamics and transmission.
The emergence of advanced biological imaging techniques, such as 3D X-ray microcomputed tomography (micro-CT), has ushered in exciting new potential for visualizing filarial nematode stages within arthropod vectors. Micro-CT instruments use X-ray beams to irradiate targeted samples; detectors capture unabsorbed photon signals to create complex 3D renderings that specialized software programs can analyze [58, 59] (Fig. 2B). This approach facilitates the examination of filarial nematodes inside arthropod vectors with minimal physical disruption of tissues. While some sample preparation involves fixation or contrast enhancement through staining, these processes are generally less invasive than traditional dissection methods.
The utilization of 3D X-ray micro-CT, supported by histopathological analysis, has facilitated the observation of Acanthocheilonema spirocauda larvae within the fat bodies (L1) and hemocoels (L3) of seal lice (Anoplura: Echinophthirius horridus) [58, 60]. Acanthocheilonema spirocauda is a filarial nematode that primarily infects seals and sea lions as definitive hosts. Hall et al. [61] used micro-CT imaging to visualize Onchocerca L1 larvae embedded within blackfly thoracic musculature. Previous studies demonstrated the potential of micro-CT imaging to reveal the precise spatiotemporal details of infection progression within arthropod vectors [58, 60, 61]. Application of micro-CT could help reveal favorable habitats, migratory routes, and life cycle transitions of larval stages of filarial nematodes in tick vectors.
Cutting-edge molecular techniques have enabled more precise identification of nematode species and isolates transmitted by tick vectors globally (Table 1) (Fig. 2C). For example, genetic analyses have confirmed filarial nematodes in ticks such as I. ricinus [38] and R. sanguineus sensu lato (s.l.) [62,63,64]. These investigations employed molecular techniques, including DNA extraction, PCR, sequencing, and in some cases, alternative genetic assays such as metabarcoding, to identify filarial nematodes in ticks (Table 1). Molecular identification of filarial nematodes in tick vectors often relies on genetic markers such as 12S mitochondrial ribosomal RNA and cytochrome c oxidase subunit I [12, 24, 33, 34, 65]. When proper reference material is available, these markers allow accurate identification at both genus and species levels, particularly for distinguishing closely related species.
Employing PCR-based assays targeting specific filarial genes consistently facilitated the identification of prominent Onchocercidae species, such as those in the genera Cercopithifilaria, Monanema, and Acanthocheilonema, across numerous hard tick species (Table 2) [13, 62, 65,66,67]. These genetic screening techniques have enabled extensive detection of tick-borne filarial nematodes across tick vector populations, revealing the presence of pathogenic filariae spanning multiple tick genera and species. In North America, genetic analyses have enabled the discovery of filarial nematode DNA in several tick species. However, it is important to note that these molecular techniques cannot detect viable parasites or their developmental stages. There is a risk of detecting remnant DNA or recently ingested microfilariae from blood meals rather than active infections. Therefore, to confirm the biological relevance of these findings and avoid spurious detections, molecular assays should be combined with other methods, such as microscopy, 3D X-ray micro-CT, or experimental transmission studies. For example, molecular screening and high throughput sequencing (HTS) have facilitated filarial detection and species identification in I. scapularis, substantiated through multiple reports [3, 4, 13, 66]. Similar findings confirming the presence of filarial nematodes using comparable molecular techniques were also obtained in A. americanum ticks through studies by Zhang et al. [25] and Henning et al. [67] and in R. sanguineus s.l. by Lineberry et al. [33, 34].
In Europe, C. rugosicauda was initially detected in the hard tick I. ricinus through dissection and morphological studies across Austria, Germany, and France [30, 35, 50]. Later, Ramos et al. [38] used both morphological and molecular techniques, specifically dissection, microscopy, PCR, and sequencing, to detect C. rugosicauda L3 in I. ricinus nymphs from southern Italy, confirming the distribution of this nematode across Europe. Similarly, Binetruy and Duron [24] used molecular methods to detect filarial nematodes in ticks collected from French Guiana, South America. They discovered filarial species, including representatives from the genera Cercopithifilaria, Cruorifilaria, and putative Dipetalonema-related nematodes, inhabiting Amblyomma, Ixodes, and Rhipicephalus ticks in the region. Further characterization through multi-locus sequence analysis by Bruley and Duron [68] provided additional insights into tick-borne filarial diversity in this region.
There is growing interest in understanding how the tick microbiome, which refers to the diverse community of microorganisms residing within the tick, contributes to modifying tick life cycles as well as pathogen and parasite acquisition and transmission. Ticks harbor a diverse microbiome comprising endogenous and exogenous bacteria, fungi, protozoa, viruses, and filarial nematodes that can colonize tick organs [3, 55, 69]. Owing to their hematophagous lifestyle, host tissue salivation, and frequent host switching, ticks provide suitable habitats for various microbes [70]. Ticks are vectors of numerous medical and veterinary pathogens, including bacteria (such as Rickettsiales, Anaplasmataceae, and spirochetes), protozoa, and viruses, and individual ticks may be concurrently infected (coinfected) with multiple microbial pathogens and filarial nematode larvae [3, 69]. Despite the frequent occurrence of multiple infections, most investigations typically examined tick-borne microbes [71, 72] and filarial nematodes in ticks individually rather than as an integrated multi-kingdom community [14, 24, 33, 66].
Coinfection adds complexity to the tick microbiome, creating interactions that may be mutualistic, antagonistic, or manipulative between coexisting organisms [73] (Fig. 3). While studies in other arthropod vectors show that such interactions can affect transmission dynamics and host health [74, 75], relationships between tick-borne pathogens and filarial nematodes remain largely unexplored. One documented example is the association between filarial nematodes and endosymbiotic Wolbachia bacteria in tick vectors [3, 25]. Using high-throughput sequencing, Cross et al. [3] demonstrated a significant positive correlation between onchocercid filarial nematodes and Wolbachia in I. scapularis ticks from Wisconsin. Understanding these and similar relationships could provide insights into disease transmission patterns and tick physiology.
Potential interactions between tick microbiome and pathogen transmission across tick tissues. The tick microbiome, consisting of a diverse array of microorganisms acquired from the vertebrate host during blood feeding, colonizes different regions of the tick gut. These microbes can potentially influence pathogen acquisition, maintenance, and transmission through diverse biological interactions, ultimately shaping pathogen transmission dynamics from the tick vector to the vertebrate host. Understanding the complex interplay between the tick microbiome and pathogen transmission is essential for developing effective tick-borne disease control strategies. SG, salivary glands; HM, hemocoel. Illustration created on Biorender.com and adapted from [92]
Studies have documented co-occurrence of filarial nematodes with other pathogens in tick vectors. For instance, Zhang et al. [26] found filarial nematodes alongside other pathogens in A. americanum ticks, while Ramos et al. [69] and Santos et al. [55] observed C. bainae co-occurring with Hepatozoon canis in R. sanguineus s.l. However, while these studies document co-occurrence, the potential interactions between these organisms and their influence on transmission dynamics and vector competence remain to be determined.
The potential impact of filarial nematodes on tick-borne disease transmission remains poorly understood, despite the well-established role of ticks as vectors of numerous pathogens in humans and animals. This interplay between filarial nematodes and established tick-borne pathogen transmission systems presents an important area for further research. Ticks transmit a wide range of disease-causing agents, including Borrelia burgdorferi, which causes Lyme disease, Rickettsia rickettsii, which is responsible for Rocky Mountain spotted fever, and Anaplasma species, which leads to anaplasmosis [76, 77]. Key tick anatomical structures (e.g., guts, hemocoel, and salivary glands) serve as critical interfaces between the tick, its pathogens, and the vertebrate host (Fig. 3). These tissues provide distinct microenvironments potentially affecting pathogen and parasite establishment and development. While interactions between tick-borne pathogens and tick tissues have been well studied [78, 79], how filarial nematodes interact with these pathogens and tick tissues [22] requires further investigation to know the mechanism at play. These interactions likely vary with vertebrate host species, tick species, tick developmental stages and sex, and blood-feeding status (i.e., engorged versus nonengorged ticks).
As filarial nematodes migrate through tick tissues, they encounter various pathogens within the tick microbiome [80,81,82]. These interactions occur in different tick compartments, particularly in the hindgut, where many pathogens undergo critical developmental processes and transovarial transmission (i.e., the passage of pathogens from an adult female tick to her eggs). The presence of filarial nematodes during migration through these tissues may influence pathogen distribution and the local microbiome composition in ways that could affect transmission dynamics (Fig. 3). However, the specific mechanisms and consequences of these interactions remain largely unexplored.
Members of the superfamily Filarioidea (families Filariidae and Onchocercidae) parasitize primarily terrestrial vertebrate species, including mammals, birds, reptiles, and amphibians. The Onchocercidae show broader vector associations than Filariidae [45], with several genera specifically adapted to tick transmission [16]. Our current understanding of tick-borne filarial diversity and distribution remains incomplete [13, 33, 67], with five genera (Cercopithifilaria, Cherylia, Cruorifilaria, Monanema, and Yatesia) documented as using ticks as vectors (Table 3). Some of these genera may also utilize other arthropod vectors, reflecting their adaptability to different transmission routes (Table 4).
Vector competence has been experimentally shown for several filarial species through demonstrated development to infective stages (Table 5). Cercopithifilaria bainae and C. grassii develop successfully in R. sanguineus s.l., which serves as their primary vector worldwide [32, 35, 83], while C. rugosicauda shows specific adaptation to I. ricinus [30, 38]. Among Monanema species, confirmed vector relationships include M. marmotae in I. cookei, M. globulosa in Haemaphysalis leachi, and M. martini in multiple tick species (R. sanguineus s.l., R. turanicus, and Hyalomma truncatum) [18, 57, 84]. Acanthocheilonema viteae represents the only species in its genus with confirmed development in tick vectors, specifically the Ornithodoros species [51, 85, 86], although Acanthocheilonema DNA has been detected in other tick species without confirmed vector competence. Experimental studies have also demonstrated Yatesia hydrochoerus development in A. cajennense complex ticks, and C. guyanensis shows laboratory development in I. ricinus, although the latter represents a non-natural vector relationship [31, 87].
These relationships likely reflect ecological adaptations, where tick feeding behaviors—including wide host range and prolonged attachment—facilitate filarial transmission. For instance, the widespread distribution of Cercopithifilaria species appears to be facilitated by the association with cosmopolitan tick vectors such as R. sanguineus s.l. [14]. Molecular detection methods have identified additional potential tick-filarial associations awaiting confirmation of vector competence, including Cruorifilaria DNA in Amblyomma species and Monanema-related sequences in various tick species [13, 24, 25, 66, 67]. Research priorities include confirming vector competence where only molecular detection exists and investigating natural transmission patterns in endemic areas. Studies should focus on documenting complete developmental cycles in tick vectors, identifying factors that influence successful transmission, and understanding the specificity of tick-filarial relationships. Standardized methods are needed to differentiate between transient presence of filarial DNA from blood meals and established infections in tick tissues.
The transmission of filarial nematodes, particularly the well-studied genera Brugia, Dirofilaria, and Onchocerca, has been predominantly associated with insect vectors (Table 4). For example, Onchocerca species develop in black flies and biting midges [16], while Dirofilaria and Brugia species use mosquitoes as their primary vectors [88]. The apparent inability of ticks to transmit these nematodes raises intriguing questions about vector–parasite specificity and the evolutionary processes shaping these relationships. Some filarial nematodes may depend on their arthropod vectors extensively, leading to highly specialized interactions. While this dependency may provide plausible explanations for the absence of tick-borne transmission in some filarial nematodes, it is important to consider anatomical and physiological limitations. Dipterans possess distinct circulatory systems and specific tissues, such as Malpighian tubules, which are crucial for filarial larval development in some species [89]. In contrast, ticks have an open circulatory system and different organ arrangements [1]. The internal structure of ticks, lacking specific organs found in insects, may not provide the necessary anatomical environment for the development of some filarial nematodes. The feeding behavior of vectors is another critical factor. While mosquitoes and other blood-sucking insects typically feed quickly, aligning with the transmission strategy of many filarial nematodes, hard ticks feed slowly over several days [1]. This prolonged feeding pattern might be incompatible with the life cycle and transmission dynamics of some filarial species that have evolved to be transmitted during brief feeding events. The feeding duration mismatch could disrupt the delicate timing for successful filarial nematode transmission and establishment. Ticks possess distinct innate immune responses that differ from insects [90, 91]. However, the specific role of tick immunity in filarial nematode establishment and development remains to be investigated through experimental studies.
North America lacks thorough investigations of tick-borne filarial associations and transmission dynamics among endemic tick vectors and vertebrate hosts. The only known filarial nematodes documented in North America belong to the genera Acanthocheilonema, Cercopithifilaria, and Monanema (Table 2), with ixodid tick vectors implicated in transmitting these filarial nematodes to their definitive hosts. In contrast to findings from South America, Europe, Africa, Australia, and Asia, this limited evidence highlights substantial knowledge gaps in the biodiversity, host associations (both definitive and intermediate), and geographic distribution of tick-borne filarial nematodes across North America. Expanded surveillance of filarial nematodes in North American ticks is warranted to characterize the distribution and diversity of unknown tick-borne parasites and their potential tick vectors (Table 4).
This review highlights our current understanding of tick-borne filarial nematodes and identifies critical knowledge gaps. Key priorities include determining the diversity and distribution of these parasites through systematic surveillance, investigating vector competence and transmission dynamics, and understanding how coinfections with other tick-borne pathogens affect disease ecology. Research initiatives should focus on comprehensive surveys using modern detection methods, conducting experimental transmission studies, and investigating host–parasite–vector relationships. Through collaborative research efforts across disciplines, we can better understand these complex relationships and develop effective control strategies.
No datasets were generated or analyzed during the current study.
Polymerase chain reaction
Deoxyribonucleic Acid
Ribonucleic Acid
Ribosomal RNA
High throughput sequencing
Larval stages 1, 2, and 3
Microcomputed tomography
Two-dimensional
Three-dimensional
Sensu lato (in the broad sense)
Species (singular)
Species (plural)
Sonenshine DE, Roe RM. Biology of ticks (second edition). 2014. Biological Sciences Faculty Books.1. https://digitalcommons.odu.edu/biology_books/1.
de la Fuente J, Estrada-Pena A, Rafael M, Almazan C, Bermudez S, Abdelbaset AE, et al. Perception of ticks and tick-borne diseases worldwide. Pathogens. 2023;12:1258.
PubMed PubMed Central Google Scholar
Cross ST, Kapuscinski ML, Perino J, Maertens BL, Weger-Lucarelli J, Ebel GD, et al. Co-infection patterns in individual Ixodes scapularis ticks reveal associations between viral. Eukaryot Bacterial Microorgan Viruses. 2018;10:388.
Google Scholar
Tokarz R, Tagliafierro T, Sameroff S, Cucura DM, Oleynik A, Che X, et al. Microbiome analysis of Ixodes scapularis ticks from New York and Connecticut. Ticks Tick Borne Dis. 2019;10:894–900.
PubMed Google Scholar
Narasimhan S, Rajeevan N, Liu L, Zhao YO, Heisig J, Pan J, et al. Gut microbiota of the tick vector Ixodes scapularis modulate colonization of the Lyme disease spirochete. Cell Host Microbe. 2014;15:58–71.
PubMed PubMed Central Google Scholar
Shah T, Li Q, Wang B, Baloch Z, Xia X. Geographical distribution and pathogenesis of ticks and tick-borne viral diseases. Front Microbiol. 2023;14:1185829.
PubMed PubMed Central Google Scholar
Zhou H, Xu L, Shi W. The human-infection potential of emerging tick-borne viruses is a global public health concern. Nat Rev Microbiol. 2023;21:215–7.
PubMed Google Scholar
Tokarz R, Sameroff S, Tagliafierro T, Jain K, Williams SH, Cucura DM, et al. Identification of novel viruses in Amblyomma americanum, Dermacentor variabilis, and Ixodes scapularis ticks. MSphere. 2018;3:e00614-e617.
PubMed PubMed Central Google Scholar
Swanson SJ, Neitzel D, Reed KD, Belongia EA. Coinfections acquired from Ixodes ticks. Clin Microbiol Rev. 2006;19:708–27.
PubMed PubMed Central Google Scholar
Tokarz R, Jain K, Bennett A, Briese T, Lipkin WI. Assessment of polymicrobial infections in ticks in New York State. Vector-Borne and Zoonotic Dis. 2010;10:217–21.
Google Scholar
Piesman J, Mather TN, Sinsky RJ, Spielman A. Duration of tick attachment and Borrelia burgdorferi transmission. J Clin Microbiol. 1987;25:557–8.
PubMed PubMed Central Google Scholar
Bezerra-Santos MA, de Macedo LO, Nguyen VL, Manoj RR, Laidoudi Y, Latrofa MS, et al. Cercopithifilaria spp. in ticks of companion animals from Asia: new putative hosts and vectors. Ticks Tick Borne Dis. 2022;13:101957.
PubMed Google Scholar
Namrata P, Miller J, Shilpa M, Reddy P, Bandoski C, Rossi M, et al. Filarial nematode infection in Ixodes scapularis ticks collected from southern Connecticut. Vet Sci. 2014;1:5–15.
Google Scholar
Otranto D, Brianti E, Latrofa M, Annoscia G, Weigl S, Lia RP, et al. On a Cercopithifilaria sp. transmitted by Rhipicephalus sanguineus: a neglected, but widespread filarioid of dogs. Parasit Vectors. 2012;5:1.
PubMed PubMed Central Google Scholar
Lefoulon E, Bain O, Bourret J, Junker K, Guerrero R, Cañizales I, et al. Shaking the tree: multi-locus sequence typing usurps current onchocercid (filarial nematode) phylogeny. PLoS Negl Trop Dis. 2015;9:e0004233.
PubMed PubMed Central Google Scholar
Anderson RC. Nematode parasites of vertebrates. Wallingford: CABI Publishing; 2000.
Google Scholar
Wilson AJ, Morgan ER, Booth M, Norman R, Perkins SE, Hauffe HC, et al. What is a vector? Philos Trans R Soc B: Biol Sci. 2017;372:20160085.
Google Scholar
Ko RC. The transmission of Ackertia marmotae Webster, 1967 (Nematoda: Onchocercidae) of groundhogs (Marmota monax) by Ixodes cookei. Can J Zool. 1972;50:437–50.
PubMed Google Scholar
Cháves-González LE, Morales-Calvo F, Mora J, Solano-Barquero A, Verocai GG, Rojas A. What lies behind the curtain: cryptic diversity in helminth parasites of human and veterinary importance. Curr Res Parasitol Vector Borne Dis. 2022;2:100094.
PubMed PubMed Central Google Scholar
Taylor MJ, Hoerauf A, Bockarie M. Lymphatic filariasis and onchocerciasis. The Lancet. 2010;376:1175–85.
Google Scholar
Cross JH. Filarial nematodes. In: Baron S, editor. Medical Microbiology. University of Texas Medical Branch at Galveston. 1996
Bain O, Babayan S. Behaviour of filariae: morphological and anatomical signatures of their life style within the arthropod and vertebrate hosts. Filaria J. 2003;2:16.
PubMed PubMed Central Google Scholar
Gruntmeir J, Kelly M, Ramos RAN, Verocai GG. Cutaneous filarioid nematodes of dogs in the USA: are they emerging, neglected, or underdiagnosed parasites? Front Vet Sci. 2023;10:1128611.
PubMed PubMed Central Google Scholar
Binetruy F, Duron O. Molecular detection of Cercopithifilaria, Cruorifilaria and Dipetalonema-like filarial nematodes in ticks of French Guiana. Parasite. 2023;30:24.
PubMed PubMed Central Google Scholar
Zhang X, Norris DE, Rasgon JL. Distribution and molecular characterization of Wolbachia endosymbionts and filarial nematodes in Maryland populations of the lone star tick (Amblyomma americanum). FEMS Microbiol Ecol. 2011;77:50–6.
PubMed Google Scholar
Olmeda-Garcøa AS, Rodrøguez-Rodrøguez JA. Stage-specific development of a filarial nematode (Dipetalonema dracunculoides) in vector ticks. J Helminthol. 1994;68:231–5.
Google Scholar
Londono MI. Behaviour and characteristics of the filarial Dipetalonema viteae in the tick Ornithodoros tartakowskyi. Antioquia Med. 1973;23:515–6.
Google Scholar
Bain O. Recherches sur la morphogénèse des Filaires chez l’hôte intermédiaire. Ann Parasitol Hum Comp. 1972;47:251–303.
Google Scholar
Beaver PC, Burgdorfer W. A microfilaria of exceptional size from the ixodid tick, Ixodes dammini, from Shelter Island. New York J Parasitol. 1984;70:963.
PubMed Google Scholar
Untersuchungen über den Entwick- lungszyklus von Dipetalonema rugosicauda (syn. Wehrdikmansia rugosicauda) (Nematoda: Filarioidea) II. Die Entwicklung von Dipetalonema rugosicauda im Zwischenwirt Ixodes ricinus und Untersuchungen über das Vorkommen der Mikrofilarien im Reh (Capreolus capreolus). Tropenmed Parasitol. 1980;31:21–30.
PubMed Google Scholar
Yates JA, Lowrie RC. Development of Yatesia hydrochoerus (Nematoda: Filarioidea) to the infective stage in Ixodid ticks. Proc Helminthol Soc Wash. 1984;51:187–90.
Google Scholar
Bezerra-Santos MA, Dantas-Torres F, Ramos RAN. Cercopithifilaria spp. of dogs: little known but prevalent filarioids beneath the skin. Parasit Vectors. 2023;16:386.
PubMed PubMed Central Google Scholar
Lineberry MW, Sundstrom KD, Little SE, Allen KE. Molecular detection of Cercopithifilaria bainae in brown dog ticks collected from dogs across the United States. Vet Parasitol. 2021;299:109583.
PubMed Google Scholar
Lineberry MW, Sundstrom KD, Little SE, Stayton EM, Allen KE. Detection of Cercopithifilaria bainae infection in shelter dogs and ticks in Oklahoma, USA. Parasit Vectors. 2020;13:216.
PubMed PubMed Central Google Scholar
Bain O, Baker M, Chabaud AG. New data on the evolutionary line of Dipetalonema (Filarioidea. Nematoda Ann Parasitol Hum Comp. 1982;57:593–620.
PubMed Google Scholar
Brianti E, Otranto D, Dantas-Torres F, Weigl S, Latrofa MS, Gaglio G, et al. Rhipicephalus sanguineus (Ixodida, Ixodidae) as intermediate host of a canine neglected filarial species with dermal microfilariae. Vet Parasitol. 2012;10:330–7.
Google Scholar
Apanaskevich and Oliver. Life cycles and natural history of ticks. Oxford: Oxford University Press; 2003.
Google Scholar
Ramos RA, Giannelli A, Dantas-Torres F, Mallia E, Passantino G, Lia RP, et al. Cercopithifilaria rugosicauda (Spirurida, Onchocercidae) in a roe deer and ticks from southern Italy. Int J Parasitol Parasites Wildl. 2013;2:292–6.
PubMed PubMed Central Google Scholar
Boyd M, Santoro D, Craft WF, Ginn PE, Childress AL, Wellehan JFX, et al. Dermatitis caused by autochthonous Cercopithifilaria bainae from a dog in Florida, USA: clinical, histological and parasitological diagnosis and treatment. Vet Dermatol. 2018;30:68.
PubMed Google Scholar
Anderson RE. Nematode parasites of vertebrates: their development and transmission. Wallingford: CABI; 1992.
Google Scholar
Štibraniova I, Lahova M, Bartikova P. Immunomodulators in tick saliva and their benefits. Acta Virol. 2013;57:200–16.
PubMed Google Scholar
Šimo L, Kazimirova M, Richardson J, Bonnet SI. The essential role of tick salivary glands and saliva in tick feeding and pathogen transmission. Front Cell Infect Microbiol. 2017;7:281.
PubMed PubMed Central Google Scholar
Moorhouse DE. The accumulation of microfilariae beneath the sites of attachment of Ixodes tasmani. Trans R Soc Trop Med Hyg. 1969;63:22.
PubMed Google Scholar
Otranto D, Brianti E, Abramo F, Gaglio G, Napoli E, Latrofa MS, et al. Cutaneous distribution and localization of Cercopithifilaria sp. microfilariae in dogs. Vet Parasitol. 2012;190:143–50.
PubMed Google Scholar
Lok J, Walker E, Glen S. Medical Entomology: A Textbook on Public Health and Veterinary Problems. 2004; Chapter. 9.
Francischetti Ivo MB. The role of saliva in tick feeding. Front Biosci. 2009;14:2051–88.
PubMed Central Google Scholar
Neelakanta G, Sultana H. Tick saliva and salivary glands: what do we know so far on their role in arthropod blood feeding and pathogen transmission. Front Cell Infect Microbiol. 2022;11:816547.
PubMed PubMed Central Google Scholar
Uni S, Bain O, Fujita H, Matsubayashi M, Fukuda M, Takaoka H. Infective larvae of Cercopithifilaria spp (Nematoda: Onchocercidae) from hard ticks (Ixodidae) recovered from the Japanese serow (Bovidae). Parasite. 2013;20:1.
PubMed PubMed Central Google Scholar
Anteson RK. Biological studies of Monanema marmotae (Webster 1967) a filarioid parasite of the woodchuck Marmota monax canadensis. Storrs, Connecticut: University of Connecticut; 1968.
Google Scholar
Böhm LK, Supperer R. Beobachtungen über eine neue Filarie (Nematoda) Wehrdikmansia rugosicauda Böhm & Supperer 1953, aus dem subkutanen Bindegewebe des Rehes. Berlin: Springer; 1953.
Google Scholar
Baltazard M, Chabaud AG, Minou A. Cycle evolutif d’une filaire parasite de merion. C R Hebd Seances Acad Sci. 1952;234:2115–7.
PubMed Google Scholar
Frank W. Biologie von Macdonaldius oschei (Filarioidea, Onchocercinae), zugleich ein Beitrag uber die Wirtsspezifitat von Ornithodoros talaje (Ixodea) Argasidae). Zeitschrift fr Parasitenkunde. 1962;22:107–8.
Google Scholar
Bain O, Casiraghi M, Martin C, Uni S. The nematoda Filarioidea: critical analysis linking molecular and traditional approaches. Parasite. 2008;15:342–8.
PubMed Google Scholar
Patton TG, Dietrich G, Brandt K, Dolan MC, Piesman J, Gilmore RD. Saliva, salivary gland, and hemolymph collection from Ixodes scapularis ticks. J Vis Exp. 2012;60:3894.
Google Scholar
Santos MAB, de Macedo LO, Otranto D, Ramos CADN, Rêgo AGOD, Giannelli A, et al. Screening of Cercopithifilaria bainae and Hepatozoon canis in ticks collected from dogs of Northeastern Brazil. Acta Parasitol. 2018;63:605–8.
PubMed Google Scholar
Spratt DM, Haycock P. Aspects of the life history of Cercopithifilaria johnstoni (Nematoda:Filarioidea). Int J Parasitol. 1988;18:1087–92.
PubMed Google Scholar
Petit G, Bain O, Carrat C, Marval F. Development of the filaria Monanema martini in the epidermis of ixodid ticks. Ann Parasitol Hum Comp. 1988;63:54–63.
PubMed Google Scholar
Ebmer D, Handschuh S, Schwaha T, Rubio-García A, Gärtner U, Glösmann M, et al. Novel 3D in situ visualization of seal heartworm (Acanthocheilonema spirocauda) larvae in the seal louse (Echinophthirius horridus) by X-ray microCT. Sci Rep. 2022;12:14181.
Google Scholar
O’Sullivan JDB, Behnsen J, Starborg T, MacDonald AS, Phythian-Adams AT, Else KJ, et al. X-ray micro-computed tomography (μCT): an emerging opportunity in parasite imaging. Parasitology. 2018;145:848–54.
PubMed Google Scholar
Leidenberger S, Harding K, Härkönen T. Phocid seals, seal lice and heartworms: a terrestrial host-parasite system conveyed to the marine environment. Dis Aquat Organ. 2007;77:235–53.
PubMed Google Scholar
Hall MJR, Martin-Vega D, Clark B, Ghosh D, Rogers M, Pigoli D, et al. Micro-CT imaging of Onchocerca infection of Simulium damnosum s.l. blackflies and comparison of the peritrophic membrane thickness of forest and Savannah flies. Med Vet Entomol. 2021;35:231–8.
PubMed PubMed Central Google Scholar
Latrofa MS, Angelou A, Giannelli A, Annoscia G, Ravagnan S, Dantas-Torres F, et al. Ticks and associated pathogens in dogs from Greece. Parasit Vectors. 2017;10:301.
PubMed PubMed Central Google Scholar
Latrofa MS, Dantas-Torres F, Giannelli A, Otranto D. Molecular detection of tick-borne pathogens in Rhipicephalus sanguineus group ticks. Ticks Tick Borne Dis. 2014;5:943–6.
PubMed Google Scholar
Santos MAB, de Souza IB, de Macedo LO, et al. Cercopithifilaria bainae in Rhipicephalus sanguineus sensu lato ticks from dogs in Brazil. Ticks Tick Borne Dis. 2017;8:623–5.
PubMed Google Scholar
Otranto D, Brianti E, Dantas-Torres F, Weigl S, Latrofa MS, Gaglio G, et al. Morphological and molecular data on the dermal microfilariae of a species of Cercopithifilaria from a dog in Sicily. Vet Parasitol. 2011;182:221–9.
PubMed Google Scholar
Tokarz R, Tagliafierro T, Ian Lipkin W, Marques AR. Characterization of a Monanema nematode in Ixodes scapularis. Parasit Vectors. 2020;13:364.
Google Scholar
Henning TC, Orr JM, Smith JD, Arias JR, Rasgon JL, Norris DE. Discovery of filarial nematode DNA in Amblyomma americanum in Northern Virginia. Ticks Tick Borne Dis. 2016;7:315–8.
PubMed Google Scholar
Bruley M, Duron O. Multi-locus sequence analysis unveils a novel genus of filarial nematodes associated with ticks in French Guiana. Parasite. 2024;31:14.
PubMed PubMed Central Google Scholar
Ramos RAN, Giannelli A, Carbone D, Baneth G, Dantas-Torres F, Otranto D. Occurrence of Hepatozoon canis and Cercopithifilaria bainae in an off-host population of Rhipicephalus sanguineus sensu lato ticks. Ticks Tick Borne Dis. 2014;5:311–4.
PubMed Google Scholar
Baneth G. Tick-borne infections of animals and humans: a common ground. Int J Parasitol. 2014;44:591–6.
PubMed Google Scholar
Chicana B, Couper LI, Kwan JY, Tahiraj E, Swei A. Comparative microbiome profiles of sympatric tick species from the far-western United States. Insects. 2019;10:353.
PubMed PubMed Central Google Scholar
Greay TL, Gofton AW, Paparini A, Ryan UM, Oskam CL, Irwin PJ. Recent insights into the tick microbiome gained through next-generation sequencing. Parasit Vectors. 2018;11:12.
PubMed PubMed Central Google Scholar
Moutailler S, Valiente Moro C, Vaumourin E, Michelet L, Tran FH, Devillers E, et al. Co-infection of ticks: the rule rather than the exception. PLoS Negl Trop Dis. 2016;10:e0004539.
PubMed PubMed Central Google Scholar
Aliota MT, Chen CC, Dagoro H, Fuchs JF, Christensen BM. Filarial worms reduce plasmodium infectivity in mosquitoes. PLoS Negl Trop Dis. 2011;5:e963.
PubMed PubMed Central Google Scholar
Mellor PS, Boorman J. Multiplication of bluetongue virus in culicoides nubeculosus (Meigen) simultaneously infected with the virus and the microfilariae of Onchocerca cervicalis (Railliet & Henry). Ann Trop Med Parasitol. 1980;74:463–9.
PubMed Google Scholar
Eisen RJ, Kugeler KJ, Eisen L, Beard CB, Paddock CD. Tick-borne Zoonoses in the United States: persistent and emerging threats to human health. ILAR J. 2017;58:319–35.
PubMed Google Scholar
Sonenshine DE. Range expansion of tick disease vectors in North America: implications for spread of tick-borne disease. Int J Environ Res Public Health. 2018;15:478.
PubMed PubMed Central Google Scholar
Van Treuren W, Ponnusamy L, Brinkerhoff RJ, Gonzalez A, Parobek CM, Juliano JJ, et al. Variation in the microbiota of Ixodes ticks with regard to geography, species, and sex. Appl Environ Microbiol. 2015;81:6200–9.
PubMed PubMed Central Google Scholar
Qiu Y, Nakao R, Ohnuma A, Kawamori F, Sugimoto C. Microbial population analysis of the salivary glands of ticks; a possible strategy for the surveillance of bacterial pathogens. PLoS ONE. 2014;9:e103961.
PubMed PubMed Central Google Scholar
Couper LI, Kwan JY, Ma J, Swei A. Drivers and patterns of microbial community assembly in a Lyme disease vector. Ecol Evol. 2019;9:7768–79.
PubMed PubMed Central Google Scholar
Swei A, Kwan JY. Tick microbiome and pathogen acquisition altered by host blood meal. ISME J. 2016;11:813–6.
PubMed PubMed Central Google Scholar
Gall CA, Reif KE, Scoles GA, Mason KL, Mousel M, Noh SM, et al. The bacterial microbiome of Dermacentor andersoni ticks influences pathogen susceptibility. ISME J. 2016;10:1846–55.
PubMed PubMed Central Google Scholar
Pampiglione S, Canestri Trotti G, Marchetti S. Ritrovamento di Diptalonema grassii (Noè, 1907) in Rhipicephalus sanguineus su cane in Italia e descrizione di alcuni suoi stadi larvali. Parassitologia. 1983;25:316.
Google Scholar
Bianco AE, Muller R, Nelson GS. Biology of Monanema globulosa, a rodent filaria with skin-dwelling microfilariae. J Helminthol. 1983;57:259–78.
Google Scholar
Londono IM. Transmission of microfilariae and infective larvae of Dipetalonema viteae (Filarioidea) among vector ticks, Ornithodoros tartakowskyi (Argasidae), and loss of microfilariae in coxal fluid. J Parasitology. 1976;62:786–8.
Google Scholar
Bain O, Chabaud A, Duke BOL, Kouznetzov R, Mullet RL, Wenk P. Litosoma Wite Krepkogorskaya, 1933 (Nematoda), Proposed correction to Litosoma viteae Z.n.(S) 2203. Bull Zool Nomencl. 1978;35:51–4.
Google Scholar
Bain O, Petit G, Jacquet-viallet P, Houin R. Cherylla guyanensis n. gen., n. sp., – Filaire d’un Marsupial sud-américain, transmise par tique. Ann Parasitol Hum Comp. 1985;60:727–37.
Google Scholar
Vinnie-Siow WY, Low VL, Tan TK, Wong ML, Leong CS, Ahmad NW, et al. Identification of potential vectors of Dirofilaria immitis and Brugia pahangi (Spirurida: Filariidae): first observation of infective third-stage larva of B pahangi in Culex quinquefasciatus (Diptera: Culicidae). Pathog Glob Health. 2022;116:356–64.
PubMed PubMed Central Google Scholar
Ledesma N, Harrington L. Mosquito vectors of dog heartworm in the United States: vector status and factors influencing transmission efficiency. Top Companion Anim Med. 2011;26:178–85.
PubMed Google Scholar
Smith AA, Pal U. Immunity-related genes in Ixodes scapularis perspectives from genome information. Front Cell Infect Microbiol. 2014;4:116.
PubMed PubMed Central Google Scholar
Hajdušek O, Šíma R, Ayllón N, Jalovecká M, Perner J, de la Fuente J, et al. Interaction of the tick immune system with transmitted pathogens. Front Cell Infect Microbiol. 2013;3:26.
PubMed PubMed Central Google Scholar
Narasimhan S, Swei A, Abouneameh S, Pal U, Pedra JHF, Fikrig E. Grappling with the tick microbiome. Trends Parasitol. 2021;37:722–33.
PubMed PubMed Central Google Scholar
Uni S, Bain O, Suzuki K, Agatsuma T, Harada M, Motokawa M, et al. Acanthocheilonema delicata n. sp. (Nematoda: Filarioidea) from Japanese badgers (Meles anakuma): description, molecular identification, and Wolbachia screening. Parasitol Int. 2013;62:14–23.
PubMed Google Scholar
Vicente JJ, Rodrigues HO, Gomes DC, Pinto RM. Nematóides do Brasil. Parte V: Nematóides de mamíferos. Rev Bras Zool. 1997;14:1–452.
Google Scholar
Eberhard ML, Morales GA, Orihel TC. Cruorifilaria tuberocauda gen. et sp. n. (Nematoda: Filarioidea) from the Capybara Hydrochoerus hydrochaeris in Colombia J Parasitol. 1976;62:604.
PubMed Google Scholar
Morales GA, Guzman VH, Angel D. Vascular damage is caused by Cruorifilaria tuberocauda in the capybara (Hydrochoerus hydrochaeris). J Wildl Dis. 1978;14:15–21.
PubMed Google Scholar
Spratt DM. Monanema australe sp. nov (Nematoda: Onchocercidae) from the lungs and liver of native rodents in North Queensland. Trans R Soc S Aust. 2008;132:1–6.
Google Scholar
Webster WA. Ackertia marmotae n. sp. (Filarioidea: Onchocercinae) from the groundhog (Marmota monax). Can J Zool. 1967;45:277–83.
Google Scholar
Junker K, Medger K, Lutermann H, Bain O. Monanema joopin. Sp. (Nematoda, Onchocercidae) from Acomys (Acomys) spinosissimus Peters, 1852 (Muridae) in South Africa, with comments on the filarial genus. Parasite. 2012;19:331–40.
PubMed PubMed Central Google Scholar
Bain O, Bartlett C, Petit G. Une Filaire de Muridés africains dans la paroi du colon, Monanema martinin sp. Ann Parasitol Hum Comp. 1986;61:465–72.
PubMed Google Scholar
Yates JA, Jorgenson JP. Dipetalonema (Alafilaria) hydrochoerus subgen. et sp. N. (Nematoda: Filarioidea) from Colombian Capybaras. J Parasitol. 1983;69:606.
PubMed Google Scholar
Andersson MO, Chitimia-Dobler L. Detection of Cercopithifilaria bainae in western Romania. Parasitol Res. 2017;116:3235–8.
PubMed Google Scholar
Angelou A, Latrofa MS, Annoscia G, Symeonidou I, Theodoridis A, Polizopoulou ZS, et al. Cercopithifilaria species in dogs and ticks from Greece. Parasitol Res. 2020;119:3391–400.
PubMed Google Scholar
Olmeda-Garcøa AS, Rodrøguez-Rodrøguez JA. Experimental transmission of Dipetalonema dracunculoides (Cobbold 1870) by Rhipicephalus sanguineus (Latreille 1806). Vet Parasit. 1993;27:339–42.
Google Scholar
Singh DP, Chatterjee RK, Sen AB. Studies on susceptibility of Ornithodoros moubata as vector of Dipetalonema viteae (Filariodea). J Commun Dis. 1988;20:220–5.
PubMed Google Scholar
Otranto D, Brianti E, Dantas-Torres F, Miró G, Latrofa MS, Mutafchiev Y, et al. Species diversity of dermal microfilariae of the genus Cercopithifilaria infesting dogs in the Mediterranean region. Parasitology. 2013;140:99–108.
PubMed Google Scholar
Bain O, Petit G, Gueye A. Transmission expérimentale de Monanema nilotica El Bihari et coll., 1977, filaire à microfilaires dermiques parasite de Muridés africains [Experimental transmission of Monanema nilotica El Bihari & coll., 1977, a filaria with skin-dwelling microfilaria parasitic in African murids]. Ann Parasitol Hum Comp. 1985;60:83–9.
PubMed Google Scholar
Dantas-Torres F, de Sousa-Paula LC, Otranto D. The Rhipicephalus sanguineus group updated list of species geographical distribution, and vector competence. Parasit Vectors. 2024;17:540.
PubMed PubMed Central Google Scholar
Download references
The authors thank Drs. Sarah Hamer and Gabriel Hamer of Texas A&M University for their inspiration and insight in writing this review manuscript.
Not applicable.
Ecology and Evolutionary Biology Program, Texas A&M University, College Station, TX, USA
Oluwaseun D. Ajileye & Jessica E. Light
Department of Ecology and Conservation Biology, Texas A&M University, College Station, TX, USA
Oluwaseun D. Ajileye & Jessica E. Light
Department of Veterinary Pathobiology, College of Veterinary Medicine and Biomedical Sciences, Texas A&M University, College Station, TX, USA
Guilherme G. Verocai
You can also search for this author in PubMed Google Scholar
You can also search for this author in PubMed Google Scholar
You can also search for this author in PubMed Google Scholar
O.D.A. and J.E.L. conceived the review ideas, and O.D.A. wrote the manuscript; J.E.L. and G.G.V. guided the manuscript writing process. All authors read and approved the final draft of the manuscript.
Correspondence to Oluwaseun D. Ajileye or Jessica E. Light.
Not applicable.
Not applicable.
The authors declare no competing interests.
Springer Nature remains neutral with regard to jurisdictional claims in published maps and institutional affiliations.
Open Access This article is licensed under a Creative Commons Attribution 4.0 International License, which permits use, sharing, adaptation, distribution and reproduction in any medium or format, as long as you give appropriate credit to the original author(s) and the source, provide a link to the Creative Commons licence, and indicate if changes were made. The images or other third party material in this article are included in the article’s Creative Commons licence, unless indicated otherwise in a credit line to the material. If material is not included in the article’s Creative Commons licence and your intended use is not permitted by statutory regulation or exceeds the permitted use, you will need to obtain permission directly from the copyright holder. To view a copy of this licence, visit http://creativecommons.org/licenses/by/4.0/. The Creative Commons Public Domain Dedication waiver (http://creativecommons.org/publicdomain/zero/1.0/) applies to the data made available in this article, unless otherwise stated in a credit line to the data.
Reprints and permissions
Ajileye, O.D., Verocai, G.G. & Light, J.E. A review of filarial nematodes parasitizing tick vectors: unraveling global patterns in species diversity, host associations, and interactions with tick-borne pathogens. Parasites Vectors 18, 50 (2025). https://doi.org/10.1186/s13071-025-06690-6
Download citation
Received:
Accepted:
Published:
DOI: https://doi.org/10.1186/s13071-025-06690-6
Anyone you share the following link with will be able to read this content:
Sorry, a shareable link is not currently available for this article.
Provided by the Springer Nature SharedIt content-sharing initiative
Advertisement
ISSN: 1756-3305
By using this website, you agree to our Terms and Conditions, Your US state privacy rights, Privacy statement and Cookies policy. Your privacy choices/Manage cookies we use in the preference centre.
© 2025 BioMed Central Ltd unless otherwise stated. Part of Springer Nature.